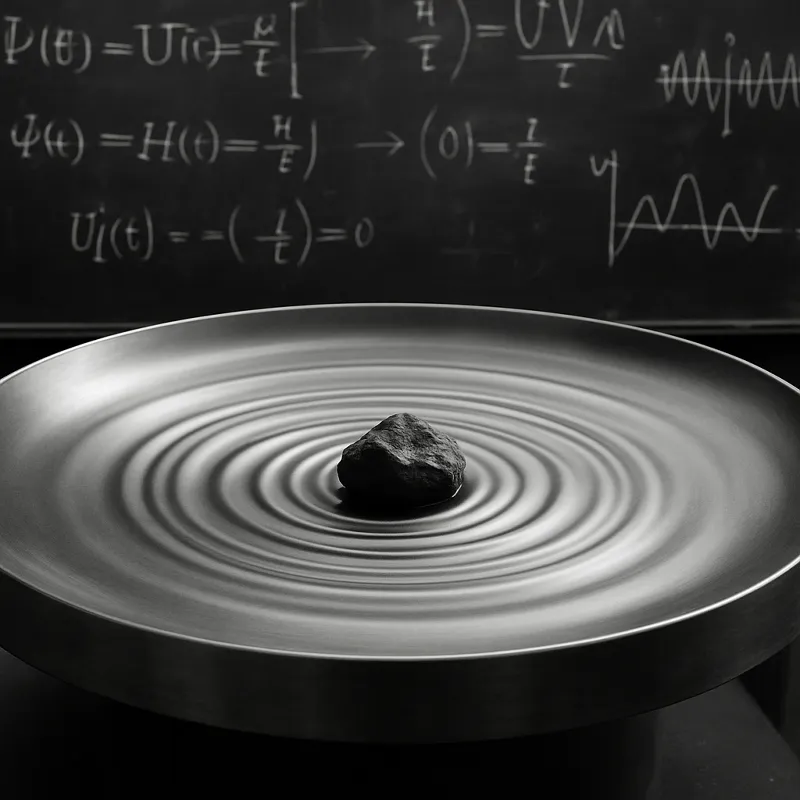
Understanding Quantum Quenches
Quantum quenches might sound like a term from a sci-fi movie, but it’s a fascinating concept in the world of physics. Imagine a world where everything is in a state of perfect balance, like a calm lake on a windless day. In quantum physics, this balance is known as equilibrium. Now, picture throwing a rock into that calm lake. The ripples that form are akin to what happens during a quantum quench. Essentially, a quantum quench involves suddenly changing a parameter in a quantum system, which throws it out of equilibrium. This sudden change can be compared to turning off the lights in a room and then suddenly turning them back on, causing a momentary confusion before the eyes adjust.
Why Quantum Quenches Matter
Quantum quenches are more than just a theoretical curiosity. They offer insight into the fundamental behavior of quantum systems, which are the building blocks of the universe. These systems are governed by the strange and counterintuitive rules of quantum mechanics. Understanding how they respond to changes can help scientists develop new technologies, such as quantum computers and advanced materials. Furthermore, studying quantum quenches can lead to a deeper understanding of how the universe behaves at its most fundamental level.
The Process of Thermalization
After a quantum quench, quantum systems do not remain in disarray forever. Much like how the ripples in a lake eventually settle down, quantum systems tend to reach a new state of equilibrium over time. This process is known as thermalization. Imagine a group of children on a playground. At first, they run around chaotically, but eventually, they settle into a game or activity. Similarly, after a quantum quench, the particles in a system interact and exchange energy until they reach a stable state that resembles thermal equilibrium.
Importance of Thermalization
Thermalization is crucial because it explains how quantum systems transition from disorder back to order. This process helps scientists understand how energy is distributed among particles in a system and how this distribution affects the system’s properties. By studying thermalization, researchers can gain insights into the fundamental laws that govern the universe and apply this knowledge to practical applications, such as improving the efficiency of energy systems or developing new materials with unique properties.
Non-thermal States
Not all systems that undergo a quantum quench reach thermal equilibrium. Some systems can get stuck in non-thermal states, which do not conform to the usual rules of thermalization. Picture a game of musical chairs where some children refuse to sit even when the music stops. These non-thermal states are intriguing because they challenge our understanding of how quantum systems behave. They are characterized by persistent oscillations or patterns that do not dissipate over time, offering a glimpse into complex quantum phenomena that are still not fully understood.
Experiments and Observations
Scientists use advanced experiments to observe quantum quenches and thermalization in action. These experiments often involve trapping atoms or molecules in controlled environments and manipulating them with lasers or magnetic fields. By observing how these systems react to sudden changes, researchers can test theoretical predictions and gain insight into the underlying mechanics of quantum systems. For example, ultracold atomic gases have been used extensively to study quantum quenches, as they can be precisely controlled and offer a clean environment for observing quantum behavior.
Real-world Applications
The study of quantum quenches and thermalization is not just an academic exercise. It has the potential to revolutionize technology and industry. For instance, understanding how quantum systems respond to changes can lead to the development of more efficient energy transfer systems, such as solar panels and batteries. Additionally, insights from these studies can aid in the creation of quantum computers, which promise to solve complex problems that are currently beyond the reach of classical computers. These applications highlight the practical significance of fundamental research in quantum physics.
Challenges and Future Directions
Despite significant progress, many challenges remain in the study of quantum quenches and thermalization. One major challenge is the complexity of quantum systems, which can behave unpredictably and are difficult to model accurately. Additionally, non-thermal states present a puzzle that scientists are eager to solve. Future research aims to develop better theoretical models and experimental techniques to overcome these challenges. By doing so, scientists hope to unlock new possibilities in quantum technology and deepen our understanding of the universe.
Conclusion
Quantum quenches and thermalization are fascinating areas of study that bridge the gap between theoretical physics and practical applications. By understanding how quantum systems respond to sudden changes and eventually reach equilibrium, scientists can gain valuable insights into the fundamental principles that govern the universe. This knowledge has the potential to drive technological advancements and open new frontiers in quantum research, making it an exciting field with implications for the future of science and technology.
관련 글: Cutoff Dependence and Matching Conditions in Effective Field Theory